
spatial disorientation
Defines our natural ability to maintain our body orientation and/or
posture in relation to the surrounding environment (physical space) at
rest and during motion. Genetically speaking, humans are designed to
maintain spatial orientation on the ground. The three-dimensional
environment of flight is unfamiliar to the human body, creating sensory
conflicts and illusions that make spatial orientation difficult, and
sometimes impossible to achieve. Statistics show that between 5 to 10% of
all general aviation accidents can be attributed to spatial
disorientation, 90% of which are fatal.
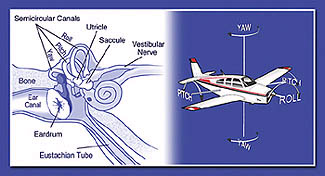
Spatial Orientation in Flight
Spatial orientation in flight is difficult to achieve because numerous
sensory stimuli (visual, vestibular, and proprioceptive) vary in
magnitude, direction, and frequency. Any differences or discrepancies
between visual, vestibular, and proprioceptive sensory inputs result in a
sensory mismatch that can produce illusions and lead to spatial
disorientation. Good spatial orientation relies on the effective
perception, integration and interpretation of visual, vestibular (organs
of equilibrium located in the inner ear) and proprioceptive (receptors
located in the skin, muscles, tendons, and joints) sensory information.
Vestibular Aspects
of Spatial Orientation
The inner ear contains the vestibular system, which is also known as the
organ of equilibrium. About the size of an pencil eraser, the vestibular
system contains two distinct structures: the semicircular canals, which
detect changes in angular acceleration, and the otolith organs (the
utricule and the saccule), which detect changes in linear acceleration and
gravity. Both the semicircular canals and the otolith organs provide
information to the brain regarding our body’s position and movement. A
connection between the vestibular system and the eyes helps to maintain
balance and keep the eyes focused on an object while the head is moving or
while the body is rotating.
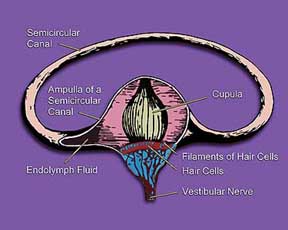
The Semicircular Canals
The semicircular canals are three half-circular, interconnected tubes
located inside each ear that are the equivalent of three gyroscopes
located in three planes perpendicular (at right angles) to each other.
Each plane corresponds to the rolling, pitching, or yawing motions of an
aircraft.
Each canal is filled with a fluid called endolymph and contains a motion
sensor with little hairs whose ends are embedded in a gelatinous structure
called the cupula. The cupula and the hairs move as the fluid moves inside
the canal in response to an angular acceleration.
The
movement of the hairs is similar to the movement of seaweed caused by
ocean currents or that of wheat fields moved by wind gusts. When the head
is still and the airplane is straight and level, the fluid in the canals
does not move and the hairs stand straight up, indicating to the brain
that there is no rotational acceleration (a turn).
If you turn either your aircraft or your head, the canal moves with your
head, but the fluid inside does not move because of its inertia. As the
canal moves, the hairs inside also move with it and are bent in the
opposite direction of the acceleration by the stationary fluid (A). This
hair movement sends a signal to the brain to indicate that the head has
turned. The problem starts when you continue turning your aircraft at a
constant rate (as in a coordinated turn) for more than 20 seconds. In this
kind of turn, the fluid inside the canal starts moving initially, then
friction causes it to catch up with the walls of the rotating canal (B).
When this happens, the hairs inside the canal will return to their
straight up position, sending an erroneous signal to the brain that the
turn has stopped–when, in fact, the turn continues. If you then start
rolling out of the turn to go back to level flight, the fluid inside the
canal will continue to move (because of its inertia), and the hairs will
now move in the opposite direction (C), sending an erroneous signal to the
brain indicating that you are turning in the opposite direction, when in
fact, you are actually slowing down from the original turn.
Vestibular Illusions
(Somatogyral - Semicircular Canals)
Illusions involving the semicircular canals of the vestibular system occur
primarily under conditions of unreliable or unavailable external visual
references and result in false sensations of rotation. These include the
Leans, the Graveyard Spin and Spiral, and the Coriolis Illusion.
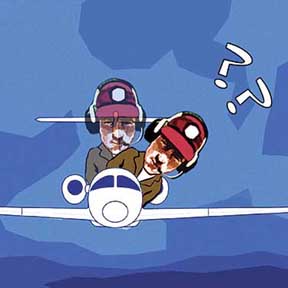
The Leans.
This is the most common illusion during flight and is caused by a sudden
return to level flight following a gradual and prolonged turn that went
unnoticed by the pilot. The reason a pilot can be unaware of such a
gradual turn is that human exposure to a rotational acceleration of 2
degrees per second or lower is below the detection threshold of the
semicircular canals. Levelling the wings after such a turn may cause an
illusion that the aircraft is banking in the opposite direction. In
response to such an illusion, a pilot may lean in the direction of the
original turn in a corrective attempt to regain the perception of a
correct vertical posture.
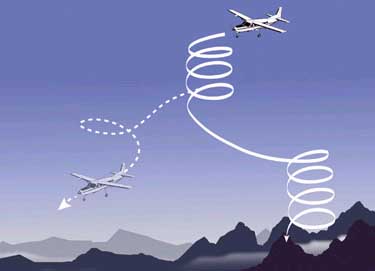
The Graveyard
Spin is an illusion that can occur to a pilot who intentionally
or unintentionally enters a spin. For example, a pilot who enters a spin
to the left will initially have a sensation of spinning in the same
direction. However, if the left spin continues the pilot will have the
sensation that the spin is progressively decreasing. At this point, if the
pilot applies right rudder to stop the left spin, the pilot will suddenly
sense a spin in the opposite direction (to the right). If the pilot
believes that the airplane is spinning to the right, the response will be
to apply left rudder to counteract the sensation of a right spin. However,
by applying left rudder the pilot will unknowingly re-enter the original
left spin. If the pilot cross checks the turn indicator, he/she would see
the turn needle indicating a left turn while he/she senses a right turn.
This creates a sensory conflict between what the pilot sees on the
instruments and what the pilot feels. If the pilot believes the body
sensations instead of trusting the instruments, the left spin will
continue. If enough altitude is lost before this illusion is recognized
and corrective action is taken, impact with terrain is inevitable.
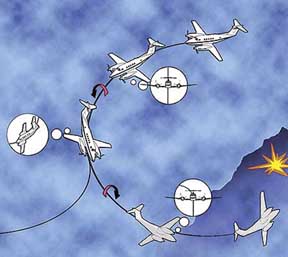
The Graveyard
Spiral is more common than the Graveyard Spin, and it is
associated with a return to level flight following an intentional or
unintentional prolonged bank turn. For example, a pilot who enters a
banking turn to the left will initially have a sensation of a turn in the
same direction. If the left turn continues (~20 seconds or more), the
pilot will experience the sensation that the airplane is no longer turning
to the left. At this point, if the pilot attempts to level the wings this
action will produce a sensation that the airplane is turning and banking
in the opposite direction (to the right). If the pilot believes the
illusion of a right turn (which can be very compelling), he/she will
re-enter the original left turn in an attempt to counteract the sensation
of a right turn. Unfortunately, while this is happening, the airplane is
still turning to the left and losing altitude. Pulling the control
yoke/stick and applying power while turning would not be a good
idea–because it would only make the left turn tighter. If the pilot fails
to recognize the illusion and does not level the wings, the airplane will
continue turning left and losing altitude until it impacts the ground.
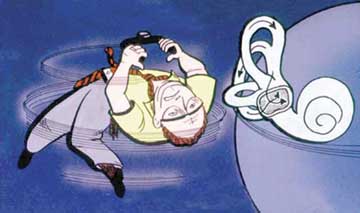
The Coriolis
Illusion involves the simultaneous stimulation of two
semicircular canals and is associated with a sudden tilting (forward or
backwards) of the pilot’s head while the aircraft is turning. This can
occur when you tilt you head down (to look at an approach chart or to
write a note on your knee pad), or tilt it up (to look at an overhead
instrument or switch) or tilt it sideways. This produces an almost
unbearable sensation that the aircraft is rolling, pitching, and yawing
all at the same time, which can be compared with the sensation of rolling
down on a hillside. This illusion can make the pilot quickly become
disoriented and lose control of the aircraft.
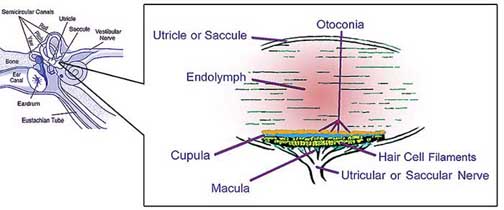
Two otolith organs, the
saccule and utricle, are located in each ear and are set at right angles
to each other. The utricle detects changes in linear acceleration in the
horizontal plane, while the saccule detects gravity changes in the
vertical plane. However, the inertial forces resulting from linear
accelerations cannot be distinguished from the force of gravity;
therefore, gravity can also produce stimulation of the utricle and saccule.
These organs are located at the base (vestibule) of the semicircular
canals, and their structure consists of small sacs (maculas) covered by
hair cell filaments that project into an overlying gelatinous membrane (cupula)
tipped by tiny, chalk-like calcium stones called otoconia.
Change in Gravity
When the head is tilted, the weight of the otoconia of the saccule pulls
the cupula, which in turn bends the hairs that send a signal to the brain
indicating that the head has changed position. A similar response will
occur during a vertical take-off in a helicopter or following the sudden
opening of a parachute after a free fall.
Change in Linear Acceleration
The inertial forces resulting from a forward linear acceleration
(take-off, increased acceleration during level flight, vertical climb)
produce a backward displacement of the otoconia of the utricle that pulls
the cupula, which in turn bends the hair cell filaments that send a signal
to the brain, indicating that the head and body have suddenly been moved
forward. Exposure to a backward linear acceleration, or to a forward
linear deceleration has the opposite effect.
Vestibular
Illusions
(Somatogravic - Utricle and Saccule)
Illusions involving the utricle and the saccule of the vestibular system
are most likely under conditions with unreliable or unavailable external
visual references. These illusions include: the Inversion Illusion,
Head-Up Illusion, and Head-Down Illusion.
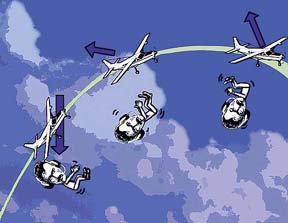
The Inversion
Illusion involves a steep ascent (forward linear acceleration) in
a high-performance aircraft, followed by a sudden return to level flight.
When the pilot levels off, the aircraft’ speed is relatively higher. This
combination of accelerations produces an illusion that the aircraft is in
inverted flight. The pilot’s response to this illusion is to lower the
nose of the aircraft.
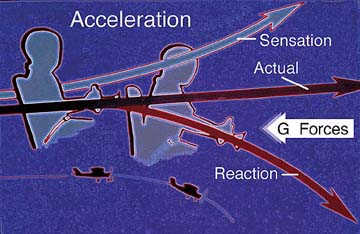
The Head-Up
Illusion involves a sudden forward linear acceleration during
level flight where the pilot perceives the illusion that the nose of the
aircraft is pitching up. The pilot’s response to this illusion would be to
push the yolk or the stick forward to pitch the nose of the aircraft down.
A night take-off from a well-lit airport into a totally dark sky (black
hole) or a catapult take-off from an aircraft carrier can also lead to
this illusion, and could result in a crash.
The Head-Down
Illusion involves a sudden linear deceleration (air braking,
lowering flaps, decreasing engine power) during level flight where the
pilot perceives the illusion that the nose of the aircraft is pitching
down. The pilot’s response to this illusion would be to pitch the nose of
the aircraft up. If this illusion occurs during a low-speed final
approach, the pilot could stall the aircraft.
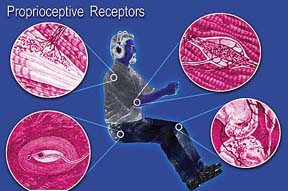
The
Proprioceptive Receptors
The proprioceptive receptors (proprioceptors) are special sensors located
in the skin, muscles, tendons, and joints that play a very small role in
maintaining spatial orientation in normal individuals. Proprioceptors do
give some indication of posture by sensing the relative position of our
body parts in relation to each other, and by sensing points of physical
contact between body parts and the surrounding environment (floor, wall,
seat, arm rest, etc.). For example, proprioceptors make it possible for
you to know that you are seated while flying; however, they alone will not
let you differentiate between flying straight and level and performing a
coordinated turn.
How to Prevent Spatial Disorientation
The following are basic steps that should help prevent spatial
disorientation:
Take the opportunity to
experience spatial disorientation illusions in a Barany chair, a Vertigon,
a GYRO, or a Virtual Reality Spatial Disorientation Demonstrator.
-
Before flying with less than 3 miles visibility, obtain training and
maintain proficiency in airplane control by reference to instruments
-
When
flying at night or in reduced visibility, use the flight instruments.
-
If
intending to fly at night, maintain night-flight currency. Include
cross-country and local operations at different airports.
-
If
only Visual Flight Rules-qualified, do not attempt visual flight when
there is a possibility of getting trapped in deteriorating weather.
-
If
you experience a vestibular illusion during flight, trust your
instruments and disregard your sensory perceptions.
Spatial Disorientation and Airsickness
It is important to know the difference between spatial disorientation and
airsickness. Airsickness is a normal response of healthy individuals when
exposed to a flight environment characterized by unfamiliar motion and
orientation clues. Common signs and symptoms of airsickness include:
vertigo, loss of appetite, increased salivation and swallowing, burping,
stomach awareness, nausea, retching, vomiting, increased need for bowel
movements, cold sweating, skin pallor, sensation of fullness of the head,
difficulty concentrating, mental confusion, apathy, drowsiness, difficulty
focusing, visual flashbacks, eye strain, blurred vision, increased
yawning, headache, dizziness, postural instability, and increased fatigue.
The symptoms are usually progressive. First, the desire for food is lost.
Then, as saliva collects in the mouth, the person begins to perspire
freely, the head aches, and the airsick person may eventually become
nauseated and vomit. Severe airsickness may cause a pilot to become
completely incapacitated.
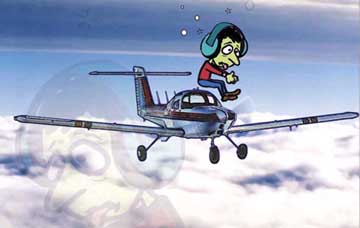
Although airsickness is uncommon among experienced pilots, it does occur
occasionally (especially among student pilots). Some people are more
susceptible to airsickness than others. Fatigue, alcohol, drugs,
medications, stress, illnesses, anxiety, fear, and insecurity are some
factors that can increase individual susceptibility to motion sickness of
any type. Women have been shown to be more susceptible to motion sickness
than men of any age. In addition, reduced mental activity (low mental
workload) during exposure to an unfamiliar motion has been implicated as a
predisposing factor for airsickness. A pilot who concentrates on the
mental tasks required to fly an aircraft will be less likely to become
airsick because his/her attention is occupied. This explains why sometimes
a student pilot who is at the controls of an aircraft does not get
airsick, but the experienced instructor who is only monitoring the student
unexpectedly becomes airsick.
A pilot who has been the victim of airsickness knows how uncomfortable and
impairing it can be. Most importantly, it jeopardizes the pilot’s flying
proficiency and safety, particularly under conditions that require peak
piloting skills and performance (equipment malfunctions, instrument flight
conditions, bad weather, final approach, and landing).
Pilots who are susceptible to airsickness should not take anti-motion
sickness medications (prescription or over-the-counter). These medications
can make one drowsy or affect brain functions in other ways. Research has
shown that most anti-motion sickness medications cause a temporary
deterioration of navigational skills or other tasks demanding keen
judgment.
An effective method to increase pilot resistance to airsickness consists
of repetitive exposure to the flying conditions that initially resulted in
airsickness. In other words, repeated exposure to the flight environment
decreases an individual’s susceptibility to subsequent airsickness.
If you become airsick while piloting an aircraft, open the air vents,
loosen your clothing, use supplemental oxygen, keep your eyes on a point
outside the aircraft, place your head against the seat’s headrest, and
avoid unnecessary head movements. Then, cancel the flight, and land as
soon as possible.
|