hazards encountered in and near thunderstorms
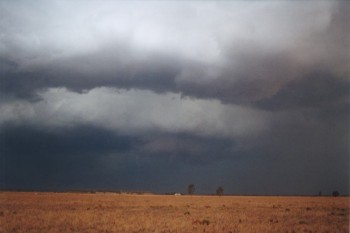
turbulence
The turbulence associated with clouds types is:
St
slight
Ci, Cs, Cc, Ac, As
nil or slight except when Ac cas or when merging into Cb
Sc
moderate
Ns
moderate but may be severe near base
Cu, TCu, Cb
Generally severe but may be catastrophic and include the downbursts
described below and the internal up/downdraughts.
Thunderstorms may be classified
in four generalised types single-cell, isolated multicell cluster, multicell
squall line (refer 9.6 below) and supercell, although supercells are also
multi-cellular. Their associated surface winds may be both high velocity and
extremely turbulent, originating from the downdraughts of cold, dense air.
When thunderstorms are about light aircraft should not be airborne.
Single-cell storms are usually isolated storms moving with the mid-level
wind, common in summer and occurring in conditions where the wind velocity,
relative to the cell motion, does not change markedly with height. (Cb
development has to be strong to overcome the detrimental effects of vertical
wind shear). A single cell storm may last less than 30 minutes, its life being
limited to the growth and collapse of a single updraught pulse. The diameter of
the storm may be less than one nautical mile and it will not move very far in
its lifetime less than 3 nm in light winds. Such storms do not usually produce
violent wind shear near the surface although microbursts may descend from even a
mild looking Cb.
Isolated single cell storms, embedded in low level cloud layers, commonly form
in cold winter air streams. They are generally frequent, but short-lived, with
soft hail and shallow wind gusts, and caused by de-stabilisation of the cold air
mass. They can be accentuated by orographic effects.
Multicell cluster storms, the most common, consist of a series of
updraught pulses (cells) which may be separated by time and/or distance and be
closely or widely spaced but moving as a single unit. They may cycle through
strong and weak phases, strength being indicated by closeness of pulses.
Frontal, pre-frontal, heat-trough and convergence zone systems may produce very
vigorous storms several miles wide and, by continually propagating new cells,
last an hour or more before the cool outflow finally undercuts and chokes off,
or smothers, the warm inflow and the system collapses. Each new cell is usually
formed in the zone of maximum convergence where the gust front directly
opposes the low level wind.
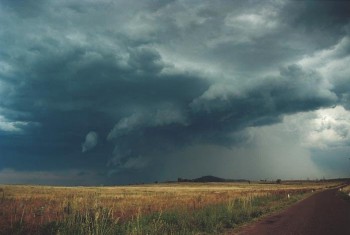
Weaker multicell storms advance with or to the left of the prevailing mid-level
wind ( i.e. that about base height plus one third of the cloud depth) at
an average rate of 10 knots or so, but the strongest storms may turn almost at
right angles to the wind. Cells will move with the prevailing mid-level wind but
because of the wind change with height the new cells form on the left, if the
wind backs with height, and on the right if it veers. Thus the storm turns
towards the flank where the new updraughts are building the flanking line,
which is a line of Cu or TCu stepped up to the most active cell. If the new
cells are forming on the upwind side, usually to the west or north-west a
back-building storm it may appear to move slowly, possibly staying in one
place for considerable time.
Strong updraught / weak downdraught storms often form in conditions where there
is moist air at most levels. Such storms produce heavy rain and may produce
severe hail but, because of the lack of dry air inflow, severe low level shear
is unlikely.
In severe storms, with strong updraughts and downdraughts, updraught velocities
increase with height, typically 1500 feet per minute at 5000 feet and 3000 feet
per minute at 20 000 feet. Updraughts of 5000 feet per minute in the upper part
of a storm are not unusual. Downdraught velocities tend to be slightly less at
corresponding altitudes. Vertical acceleration loads of 2g to 3g may be
experienced in horizontal flight.
The areas which most concern light aircraft are the low level outflow regions
where downburst gusts of 50 knots plus may be reached in the line squall. The
spreading density current of the outflow may last for 10 to 30 minutes and be
1500 to 6000 feet deep, forcing the warm, moist low level air up and so
continuously regenerating the updraught. Thus an area up to 25 30 nm from a
large storm, and 15 25 nm for a medium storm, should be regarded as absolutely
a 'no-go' area for light aircraft. An intense narrow initial microburst may
sometimes be produced, bringing short-lived wind gusts of possibly 100 knots or
more.
There is an area of extreme low level shear at the leading edge of the storm
between the nose of the shelf cloud and where the gust front has reached,
possibly 1 3 nm ahead of the rain curtain. Shear at the trailing edge is not
quite as severe, as velocity there equals gust speed minus the speed of storm
advance.
Vertical wind shear is usually detrimental to early development of Cb cells,
however if there is:
-
strong vertical wind shear,
backing and strengthening with height,
-
associated with a deep surface
layer of warm moist air,
-
below a mid-level layer of dry
air,
-
with an inversion separating
the layers,
-
and a rapid decrease in
temperature with height above the inversion,
-
then the ideal conditions are
created for a severe multicell storm. Or a supercell storm if the surface wind
is greater than 20 knots and the vertical wind shear exceeds about five knots
for each 3000 feet.
The capping inversion
keeps the lid on development until the lifting force builds up sufficiently to
burst through the inversion and great buoyancy develops in the colder upper
layer. Upper level divergence and a jetstream will also enhance the vertical
motion.
Strong wind shear both tilts the updraught and provides the means to rotate it
(storm updraughts usually do not rotate) leading to the development of a
supercell storm. A supercell is a severe storm with a continuing,
organised strong main updraught with usually slight rotation (helicity)
and co-existing strong downdraughts, controlling and directing the inflow (
which may have a velocity of 30 50 knots) into the cell from the surrounding
atmosphere. It will usually diverge to the left of the prevailing mid-level
wind.
There may be broad anti-clockwise rotation as viewed from below of the cloud
base beneath the main updraught. Humid, rain cooled air from the downdraught may
also be pulled into the normal inflow (which is often visible as scud beneath
the Cb) causing part of the cloud base to lower, forming a circular wall cloud
at the updraught base, and if vorticity increases within the cloud, a tornadic
funnel may form. A gustnado may form under a shelf at the leading edge of the
gust front.
Broadscale rotation of a storm cell forms a mesocyclone one to ten nm in
diameter with a surface pressure drop of a few hPa at the centre although a 30
hPa drop has been recorded. Supercells may last for several hours as organised
systems and commonly form in warm, moist north / north-east flow into a surface
trough and along a dividing range during summer.
A microburst is a strong concentrated
plunge of cold dense air from a convective cloud. Peak wind gusts usually last
less than ten minutes, often 3 to 5 minutes, but extremely hazardous vertical
and horizontal shear results. It may be dry or associated with precipitation
ranging from virga showers to heavy rain showers wet. A curling outflow foot
of dust or precipitation from the surface touchdown point may be visible near
the surface.
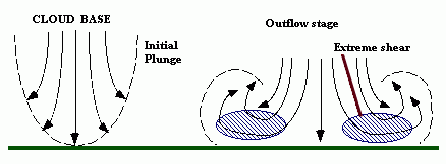
Microbursts are generally
associated with hot and relatively dry conditions at low levels (such as found
in inland Australia), convectively unstable moist air aloft with high (5000 to
15 000 feet) based Cu or TCu. If the cloud is forming when the surface
temperature/dewpoint spread is 15 °C to 25 °C then the microburst potential is
high. The high spread means the atmosphere can hold much more water vapour. Rain
falling in, and from, the cloud is evaporating (virga), thus cooling the
entrained air and resulting in downward acceleration of the denser air.
Consequently flight through, or under or near, precipitation from a large Cu
involves risk. Significant hail is unlikely. The most dangerous area is the
horizontal density current vortex ring close to the touchdown point. The ring
moves outward from the contact point at high velocity (up to 150 knots) until it
disintegrates into several horizontal roll vortices spread around the periphery
and which may continue to provide extreme shear for several minutes. The maximum
horizontal winds occur about 100 feet above ground level.
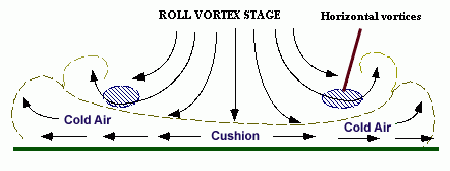
Microbursts occur under 5% 10%
of Cb (refer 9.5 below) but a less concentrated, longer lasting gust front
macroburst is normally associated with the entire cold air outflow of larger
storm cells. The severe gust fronts from a microburst extend for less than 4 km,
those from a macroburst extend for more than 4 km. The vertical gusts within the
downburst, perhaps with a velocity twice the mean, may produce a microburst
within the macroburst.
Squall
Lines
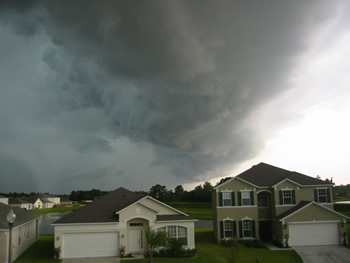
The precipitation downdraught
associated with an individual cell tends to be concentrated towards the leading
edge of the storm where the cold heavy outflow spreads out at ground level
forming a small high pressure cell, a meso-high, 10 15 nm across. The
dense air lifts the warmer, moist air in its path and may initiate a self
amplifying convective complex, in which neighbouring storm cells
consolidate into a towering squall line of large thunderstorm cells
ranged across the prevailing wind direction. At locations in the path of the
squall line the resultant line squall occurs as a sharp backing in wind
direction, severe gusts, temperature drop, hail or heavy rain and possibly
tornadoes. If the squall line is formed in an environment of strong mid-level
winds the surface gusts may exceed 50 knots.
Squall lines vary in length, some of the longest being those which develop in a
pre-frontal trough 50 -100 nm ahead of a cold front. These squall lines may be
several hundred nautical miles in length and 10 25 nm wide moving at typically
25 knots. The pre-frontal lines form ahead of the front as upper air flow
develops waves ahead of the front; downward wave flow inhibiting and upward wave
flow favouring, uplift.
During daylight hours the squall line may appear as a wall of advancing cloud
with spreading cirrus plume but the most severe effects will be close to each of
the numerous Cb cells. The convective complex releases a tremendous amount of
latent heat and moisture which may be sufficient to generate a warm core
mesoscale cyclone lasting several days.
heavy
rain
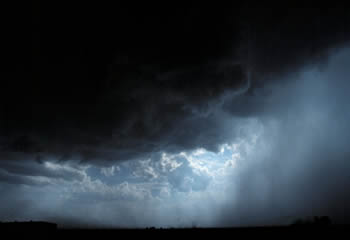
microburst with heavy rain
Flight through rain causes a
water film to form over the wings and fuselage; if the rain falls at a rate
exceeding perhaps 20 mm per hour the film over the wings is roughened by the
cratering of drop impacts and the formation of waves. The effect, which
increases with rainfall rate, is a lowering of the lift coefficient value at all
angles of attack, with laminar flow wings being most affected and fabric wings
least affected. The stall will occur at a smaller angle of attack i.e. the
stalling speed increases; which is further compounded by the increased weight of
the aircraft.
The water film will increase drag and the encounter with falling rain will apply
a downward/backward momentum which may be significant to a light aircraft.
Propeller performance is degraded and water ingestion will affect engine output.
Thus the rain effect can be hazardous when operating in conditions of low excess
aircraft energy typically when taking off, landing or conducting a go-around.
Visibility through a windscreen may be zero in such conditions, so a non IFR-equipped
aircraft will be in difficulties.
Lightning
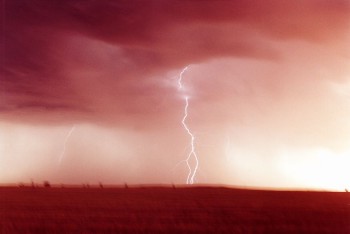
The electrostatic structure
within Cb, or Cu con, is such that pockets of different charge exist throughout
the cloud but, in 90% or more, with a main net positive charge residing on the
cloud ice crystals in the upper part of the cloud and a main net negative
charge, of similar magnitude, centred near the middle or lower part of the cloud
at the sub-freezing level, the charge mainly residing on supercooled droplets. A
smaller positive charge centre may exist at the bottom of the cloud where
temperatures are above freezing. The electrostatic forces of repulsion /
attraction induce secondary charge accumulations outside the cloud, a positive
region on the earths surface directly below the cloud. Above the cloud positive
ions are transferred away from and negative ions are transferred toward the
cloud.
One favoured theory for the charge separation mechanism is the 'precipitation'
theory which suggests that the disintegration of large raindrops and the
interaction between the smaller cloud particles and the larger precipitation
particles in the up / downdraughts causes the separation of electrical charge,
with downward motion of negatively charged cloud and precipitation particles and
upward motion of positively charged cloud particles.
Discharge channels
Lightning is a flow of current,
or discharge, along an ionized channel that equalizes the charge difference
between two regions of opposite charge, occurring when the charge potentials
exceed the electrical resistance of the intervening air. These discharges can be
between the charged regions of the same cloud (intra-cloud), between the
cloud and the ground (cloud-to-ground), between separate clouds (cloud-to-cloud)
or between the base of a cloud and a charge centre in the atmosphere underneath
it (cloud-to-air). The discharge channels, or streamers, propagate
themselves through the air by establishing, and maintaining, an avalanche effect
of free electrons which ionize atoms in their path. Lightning rates,
particularly intra-cloud strokes, increase greatly with increase in the depth of
clouds. Cloud-to-cloud and cloud-to-air discharges are rare but tend to be more
common in the high based Cb found in the drier areas of Australia. Discharges
above the Cb anvil into the stratosphere and mesosphere also occur.
When intra-cloud lightning the most common discharge occurs, it is most
often between the upper positive and the middle negative centres. The discharge
path is established by a stepped leader, the initial lightning streamer
which grows in stages and splits into more and more branches as it moves forward
seeking an optimal path between the charge centres. The second, and subsequent,
lightning strokes in a composite flash are initiated by dart leaders,
streamers which generally follow the optimum ionized channel established by the
stepped leader. The associated electrical current probably peaks at a few
thousand amperes. A distant observer cannot see the streamers but sees portion
of the cloud become luminous, for maybe less than 0.5 seconds, hence 'sheet
lightning'.
Cloud-to-ground discharges
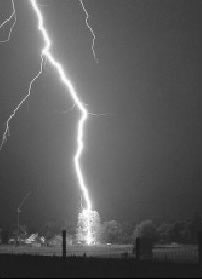
Most cloud-to-ground discharges
occur between the main negatively charged region and the surface, initially by a
stepped leader from the region which usually exhibits branching channels as it
seeks an optimal path. When the stepped leader makes contact, directly with the
earth or with a ground streamer, which is another electrical breakdown
initiated from the surface positive charge region and which rises a short
distance from the surface, the cloud is short-circuited to ground and to
complete each lightning stroke a return streamer, or return stroke,
propagates upwards. (The return streamer starts as positive ions which
capture the free electrons flowing down the channel and emit photons. The
streamer carries more positive ions upward and their interaction with the free
flowing electrons gives the impression of upwards movement.) The charge on
the branches of the stepped leader that have not been grounded flow into the
return streamer. Subsequent strokes in the composite flash are initiated by dart
leaders with a return streamer following each contact. The return streamer,
lasting 20 40 microseconds, propagates a current carrying core a few cm in
diameter with a current density of 1000 amperes per cm² and a total current
typically 20 000 amps but peaks could be much greater. A charged sheath or
corona, a few metres in diameter, exists around the core. The stroke sequence of
dart leader / return streamer occurs several times in each flash to ground,
giving it a flickering appearance. Each stroke draws charge from successively
higher regions of the Cb and transfers a negative charge to the surface. Return
streamers occur only in cloud-to-ground discharges and are so intense because of
the earths high conductivity. Some rare discharges between cloud and ground are
initiated from high surface structures or mountain peaks, by an upward moving
stepped leader and referred to as a ground-to-cloud discharge. Rather
rarely an overhanging anvil-to-ground discharge can be triggered by heavy
charge accumulation in the anvil and the high magnitude strike can move many
kilometres from the storm a 'bolt from the blue'.
The temperature of the ionised plasma in the return streamer is at least 30 000
°C and the pressure is greater than 10 atmospheres, causing supersonic expansion
of the channel which absorbs most of the dissipated energy in the flash. The
shockwave lasts for 10 20 microseconds and moves out several hundred metres
before decaying into the sound wave thunder with maximum energy at about 50
hertz. The shock wave can damage objects in its path. The channel length is
typically 5 km and channel length can be roughly determined by timing the
thunder rumble after the initial clap, e.g. a rumble lasts for 10 seconds x 335
m/sec = 3.3 km channel length. When a lightning stroke occurs within 150 m or so
the observer hears the shockwave as a single high pitched bang.
Effect on aircraft
instruments
The lightning discharges emit
radio waves atmospherics or sferics at the low end of the AM
broadcast band and at TV band 1, which are the basis for airborne storm mapping
instruments such as Stormscope and Strikefinder. The NDB/ADF navigation aids
also operate near the low end of the AM band so that the tremendous radio
frequency energy of the storm will divert the radio compass needle. Weather
radars map storms from the associated precipitation.
Strike effect on aircraft
When most aeroplanes, excluding
ultralights, are struck by lightning the streamer attaches initially to an
extremity, such as the nose or wing tip then re-attaches itself to the fuselage
at other locations as the aircraft moves through the channel. The current is
conducted through the electrically bonded aluminium skin and structures of the
aircraft and exits from an extremity, such as the tail. If an ultralight is
struck by lightning the consequences cannot be determined but are likely to be
very unpleasant. Ultralights particularly should give all Cbs a wide berth but
supercells and line squalls should be cleared by 25 30 nm at least.
Although a basic level of protection is provided in most light aeroplanes for
the airframe, fuel system and engines, damage to wing tips, propellers and
navigation lights may occur and the current has the potential to induce
transients into electrical cables or electronic equipment. The other main area
of concern is the fuel tanks, lines, vents, filler caps and their supporting
structure, where extra design precautions prevent sparking or burn through. In
heavier aircraft radomes, being constructed of non-conductive material, are at
risk.
Red sprites
and blue jets
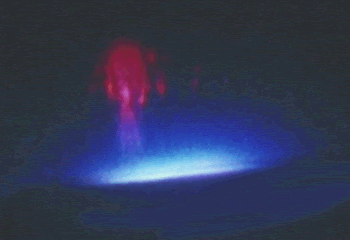
When large cloud to ground
lightning discharges occur below an extensive Cb cluster, which has a spreading
stratiform anvil, other discharges are generated above the anvil. These
discharges are in the form of flashes of light lasting just a few milliseconds
and probably not observable by the untrained, naked eye but readily recorded on
low light video.
Red sprites are very large but weak flashes of light emitted by excited
nitrogen atoms and equivalent in intensity to a moderate auroral arc. They
extend from the anvil to the mesopause at an altitude up to 90 km. The brightest
parts exist between 60 75 km, red in colour and with a faint red glow
extending above. Blue filaments may appear below the brightest region. Sprites
usually occur in clusters which may extend 50 km horizontally. Blue jets
are ejected above the Cb core and flash upward in narrow cones which fade out at
about 50 km. These optical emissions are not aligned with the local magnetic
field.
St.
Elmo's Fire
St. Elmo's fire is a plasma
(i.e. a hot, ionized gas) that forms around the tips of raised, pointed
conductors during thunderstorms. It is known as a corona discharge or
point discharge to physicists. The few people that have had the privilege of
viewing an actual St. Elmo's fire have given various descriptions. It has been
seen with different physical characteristics depending on the conditions of the
viewing. It could be blue to bluish-white, silent to emitting a hissing sound,
and ghostly to solid.
St. Elmo's fire occurs during
thunderstorms - generally after the most severe part of the storm has passed -
when the air reaches a very high voltage. These conditions are necessary to
accumulate a charge large enough to create the phenomenon. It is always
found attached to a grounded conductor with a sharp point; the most common are
masts of sailing ships, church steeples, airplane wings or propellers, or even
horns of cattle. The non-attached version of St. Elmo's fire is known as Ball
Lightning.
St. Elmo's Fire forms on
aircraft flying through heavily charged skies, often as a precursor to a
lightning strike. The glow can be seen concentrated on wing tips, antennae, the
tail, nose and propeller blades when the potential difference is large enough.
St. Elmo's Fire can be heard "singing" on the craft's radio, a frying or hissing
sound running up and down the musical scale, according to some pilots.
A British Airways 747 flight was
flying at night in the South Pacific just after a volcanic eruption of Mt.
Galunggung, in western Java. St. Elmo's fire was observed extending from the
engines and sparking across the instrument panel, while smoke was smelled inside
the passenger cabin. In minutes, the number four engine shut down, followed
quickly by the remaining three. The aircraft glided from 37,000 feet to 12,000
feet before the crew was able to restart the engines and steer for an emergency
landing at Jakarta. Ash appeared as smoke as it was sucked into the air
conditioning system. The static electricity created by the ash hitting the
windscreen and wings had created the St. Elmo's fire.
Hail
Hail can cause considerable
damage to aircraft and is usually encountered between 10,000 and 30,000 feet. At
times it can also be found in clear air near thunderstorms.
Icing
High humidity and low winter
freezing levels provide likely conditions for icing at low levels. Hopefully it
is unlikely that a VFR GA pilot would venture into possible icing conditions but
pilots may be tempted to fly through freezing rain or drizzle. Aircraft cruising
in VMC above the freezing level and then descending through a cloud layer may
pick up ice.
The prerequisites for airframe icing are:
The aircraft must be flying
through visible supercooled liquid, i.e. cloud, rain or drizzle
The airframe temperature, at
the point where the liquid strikes the surface, must be sub-zero.
The severity of icing is
dependent on the supercooled water content, the temperature and the size of the
cloud droplets or raindrops. The terms used are:
Light:
less than 0.5 grams per cubic metre of supercooled water in the cloud no
change of course or altitude is considered necessary for an aircraft equipped
to handle icing. Very few light aircraft are equipped to handle any form
of airframe ice.
vModerate:
between 0.5 and 1.0 g/m³ a diversion is desirable but the ice accretion is
insufficient to affect safety if anti-icing / de-icing used, unless flight
continued for an extended period.
Severe:
more than 1.0 g/m³ a diversion is essential. The ice accretion is continuous
and such that de-icing / anti-icing equipment will not control it and the
condition is hazardous.
The diagram below shows the ice
accretion in mm on a small probe for the air miles flown, in clouds with liquid
water content varying from 0.2 g/m³ to 1.5 g/m³.
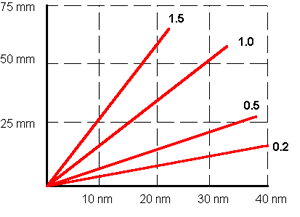
The small, supercooled droplets
in stratiform cloud tend to instantaneous freezing when disturbed and form
rime ice rough white ice, opaque with entrapped air. In the stable
conditions usually associated with stratiform cloud, icing will form where the
outside air temperature [OAT] is in the range 0 °C to 10 °C . The continuous
icing layer is usually 3000 to 4000 feet thick.
The larger supercooled droplets in convective cloud tend to freeze more slowly
when disturbed by the aircraft; spreading over the surface and forming glossy
clear or glaze ice . In unstable air moderate to severe icing may
form where the OAT is in the range 4 °C to 20 °C . Where temperature is
between 20 °C and 40 °C the chances of moderate or severe icing are small
except in CB CAL i.e newly developed cells. Icing is normally most severe
between 4 °C and 7 °C where the concentration of free supercooled droplets is
usually at maximum, i.e. the minimum number have turned to ice crystals.. Mixed
rime and clear ice can build into a heavy, rough conglomerate.
Flying through snow crystals or snowflakes will not form ice but may form a line
of heavy frosting on the wing leading edge at the point of stagnation, which
could increase stalling speed on landing. Flying through wet mushy snow, which
is a mixture of snow crystals and supercooled raindrops, will form pack snow
on the aircraft.
Ci, Cs and Cc; icing is rare
but will be light should it occur
Ac, As and St; usually light
to moderate rime
Sc; moderate rime
Ns; moderate to severe rime,
clear ice or mixed ice. As the vertical extent of Ns plus As may be 15 000 or
20 000 feet the tops of the cloud may still contain supercooled droplets at
temperatures as low as 25 °C
TCu and Cb; rime, clear or
mixed ice, possibly severe.
Freezing rain creates the worst
icing conditions, occurring when the aircraft flies through supercooled rain or
drizzle above the freezing level in Cu or Cb. The rain striking an airframe, at
sub-zero temperature, freezes and glaze ice accumulates rapidly, as much as one
cm per four miles.
Freezing rain or drizzle occurring in
clear air below the cloud base is the most likely airframe icing condition to be
encountered by the VFR pilot and, as it is unlikely to occur much above 5000
feet amsl, descent choices are possibly limited.
Effect of airframe ice
Ice accretion on the wing
leading edge is a major concern for aircraft not equipped with anti-icing or
de-icing. Airflow disruption will reduce the maximum lift coefficient attainable
by as much as 30% 50%, thus raising the stalling speed considerably. and,
because the aircraft has to fly at a greater angle of attack to maintain lift,
the induced drag also increases and the aircraft continues to lose airspeed,
making it impossible to sustain altitude if the stall is to be avoided. Fuel
consumption will also increase considerably.
The weight of 25 mm of ice on
a small GA aircraft would be about 30 to 40 kg but the increased weight is
usually a lesser problem than the change in weight distribution. Also
accretion is often not symmetrical, which adds to increasing
uncontrollability.
Forward visibility may be lost
as ice forms on the windshield.
Icing of the propeller blades
reduces thrust and may cause dangerous imbalance.
Ice may jam or restrict
control and trim surface movement or may unbalance the control surface and
possibly lead to the development of flutter.
Communication antennae may be
rendered ineffective or even snapped off.
Extension of flap may result
in rudder ineffectiveness or even increase the stalling speed.
Aircraft operating from high
altitude airfields in freezing conditions may be affected by picking up runway
snow or slush which subsequently forms ice possibly causing engine induction
icing, frozen brakes etc.
Engine air intake icing
Impact icing
may occur at the engine air intake filter. If 'alternate air' (which
draws air from within the engine cowling) is not selected, or is ineffective,
power loss will ensue. When air is near freezing movement of water molecules
over an object such as the air filter may sometimes cause instantaneous
freezing. Ice may also form on the cowling intakes and cause engine overheating.
Pitot or static vent icing
Pitot or static vent blockage
will seriously effect the ASI, VSI and altimeter, as shown in the table below,
but be aware that blockage of the static vent tubing from causes other than
icing, water for example, will render the ASI, VSI and altimeter useless, unless
the aircraft is fitted with an alternative static source.
If the static vent is totally blocked by
ice
Flight stage |
Altimeter reading |
VSI reading |
ASI reading |
During climb |
constant |
zero |
under |
During descent |
constant |
zero |
over |
During cruise |
+constant |
zero |
OK |
On take-off |
constant |
zero |
under |
If the pitot tube is totally blocked
Flight stage |
Altimeter reading |
VSI reading |
ASI reading |
During climb |
no effect |
no effect |
over* |
During descent |
no effect |
no effect |
under* |
During cruise |
no effect |
no effect |
constant* |
On take-off |
no effect |
no effect |
zero* |
If the pitot tube is partially blocked
Flight stage |
Altimeter reading |
VSI reading |
ASI reading |
During climb |
constant |
zero |
under* |
During descent |
constant |
zero |
under* |
During cruise |
+constant |
zero |
under* |
On take-off |
constant |
zero |
under* |
Very few light aircraft are
equipped for icing conditions. Devices include inflating rubber boots on the
leading edges, heating coils and surfaces that exude antifreeze. Many aircraft
however are equipped with storm scopes that give position and warning of
approaching storm cells.
Avoiding thunderstorms
a. Above all, remember this: never
regard any thunderstorm lightly, even when radar observers report the echoes are
of light intensity. Avoiding thunderstorms is the best policy. Following are
some do's and don'ts of thunderstorm avoidance:
Don't land or take off in the
face of an approaching thunderstorm. A sudden gust front of low level
turbulence could cause loss of control.
Don't attempt to fly under
a thunderstorm even if you can see through to the other side. Turbulence and windshear under the storm could be disastrous.
Don't fly without airborne
radar into a cloud mass containing scattered embedded thunderstorms. Scattered
thunderstorms not embedded usually can be visually circumnavigated.
Don't trust the visual
appearance to be a reliable indicator of the turbulence inside a thunderstorm.
Do avoid by at least 20
miles any thunderstorm identified as severe or giving an intense radar echo.
This is especially true under the anvil of a large cumulonimbus.
Do circumnavigate the
entire area if the area has 6/10 thunderstorm coverage.
Do remember that vivid and
frequent lightning indicates the probability of a severe thunderstorm.
Do regard as extremely
hazardous any thunderstorm with tops 35,000 feet or higher whether the top is
visually sighted or determined by radar.
b. If you cannot avoid
penetrating a thunderstorm, following are some do's BEFORE entering the storm:
Tighten your safety belt,
put on your shoulder harness if you have one, and secure all loose objects.
Plan and hold your course
to take you through the storm in a minimum time.
To avoid the most critical
icing, establish a penetration altitude below the freezing level or above the
level of -15 °C.
Verify that pitot heat is
on and turn on carburettor heat or jet engine anti-ice. Icing can be rapid at
any altitude and cause almost instantaneous power failure and/or loss of
airspeed indication.
Establish power settings
for turbulence penetration airspeed recommended in your aircraft manual.
Turn up cockpit lights to
highest intensity to lessen temporary blindness from lightning.
If using automatic pilot,
disengage altitude hold mode and speed hold mode. The automatic altitude and
speed controls will increase manoeuvres of the aircraft thus increasing
structural stress.
If using airborne radar,
tilt the antenna up and down occasionally. This will permit you to detect
other thunderstorm activity at altitudes other than the one being flown.
c. Following are some do's and
don'ts DURING the thunderstorm penetration:
Do keep your eyes on your
instruments. Looking outside the cockpit can increase danger of temporary
blindness from lightning.
Don't change power
settings; maintain settings for the recommended turbulence penetration
airspeed.
Do maintain constant
attitude; let the aircraft "ride the waves." Manoeuvres in trying to maintain
constant altitude increase stress on the aircraft.
Don't turn back once you
are in the thunderstorm. A straight course through the storm most likely will
get you out of the hazards most quickly. In addition, turning manoeuvres
increase stress on the aircraft.